So, seeing as I’m pre-writing this post at the moment, these finds are about a week and a half old, but no less awesome.
As of writing this, my puddle water sample from Renfree Field in Sacramento has been aging for about 4 or 5 days. Over the course of this time, the water’s temperature, lighting, pH, turbulence and bacterial population have changed enough in a well-lit corner of my bedroom-turned-microbiology lab (relative to a puddle in an outdoors parking lot) such that the normally-dominant species in nature no longer have the competitive advantage they did when I first collected my sample. What is very interesting is that this means some species which normally exist in only tiny populations in the wild then have a chance to seize the newly-vacated water column and grow to high enough densities that I actually find them. In the case of rotifers, the primary species Epiphanes senta and perhaps Asplanchna silvestrii die out to yield Brachionus of some kind. And now, as I’ve continued my observations, I’ve found another late bloomer: Polyarthra vulgaris.
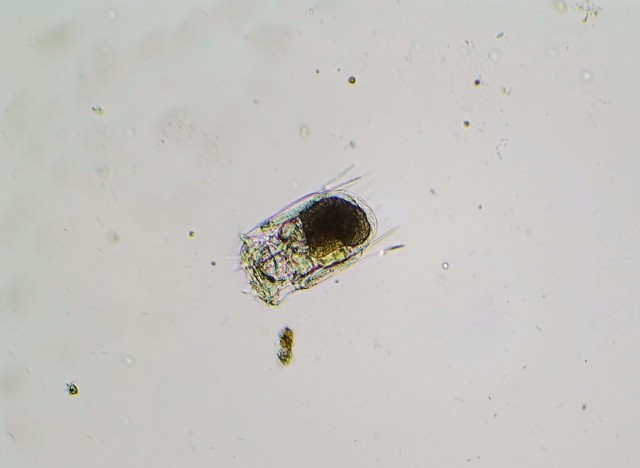
P. vulgaris is a planktonic, roughly square-shaped, soft-bodied, and relatively small rotifer in comparison to the ones I’ve previously featured on my blog – probably no more than 100 microns on average in any dimension. However, it makes up for its lack of size with a very feisty character, if one could anthropomorphize a rotifer in such a manner. This is mostly due to Polyarthra‘s unique set of swimming fins or paddles – pictured as the “spines” running down the side of the animal’s body – which it can flick very rapidly to “jump” through the water column. P. vulgaris thus has a two-speed swimming mechanism: a slow, steady and controllable cruising speed, with motion generated by the regular rotation of the cilia which the rotifer uses to eat; and the quick, random and near-instantaneous jump through the water, a result of the lighting-fast twitch of the swimming fins. Many zooplankton species exhibit these two different types of swimming, especially the species on the lower end of the food chain. The lightning jump likely developed due to a need to escape from predators (say, a hungry Asplanchna), but as it is random and costs lots of energy, the organism still uses its regular feeding motion to move around slowly and more purposefully when it is not in immediate danger. (Just another aside on the lightning jump: in a normal drop of water, these rotifers legitimately appear to teleport. That’s how fast they are.)
And here’s a slideshow of three frames I captured of the rotifer demonstrating its flicking motion:
And a video:
While the genus Polyarthra, to my knowledge, has been studied phylogenetically with DNA evidence and no doubt contains hidden species like the Epiphanes senta case, it’s still possible to identify general species “groups” based off of the visible structure of the swimming fins. Using a simple dichotomous key for zooplankton I found online, I am tentatively calling my individuals P. vulgaris due to their generally short, sleek fins. This species was first described in 1943, in a German book titled Die Plankton Rotatorien des Motalastrom: zur Taxonomic and Okologie der Plankton Rotatorien; it is a close relative of the related and longer-finned species P. dolichoptera, with which it often grows in the same body of water. The majority of research I’ve found on Polyarthra concerns its population dynamics, specifically with regards to predator-prey relationships and interspecific competition. In looking at the head-to-head comparison of the two aforementioned species of Polyarthra, P. vulgaris seems to have an advantage in warmer and more oxygen-rich waters (corresponding with the highest populations in summer and autumn), whereas P. dolichoptera prefers water up to 15 degrees Celsius cooler and can tolerate lower levels of dissolved oxygen (which explains why it often reaches highest population densities in the spring). However, both species can proliferate well in water below 10 degrees Celsius and will survive below 5.
So here’s where things get interesting.

As far as I know, P. vulgaris has been observed and collected from lakes and ponds in the northern parts of the continental United States and Europe, with one aberrant habitat being a canal in Washington. It can inhabit both oligotrophic (nutrient-poor) and eutrophic (nutrient-rich) waters, suggesting that it is relatively adaptable given its preferred temperature conditions. However, I have not come across in my reading any records of Polyarthra from ephemeral waters such as vernal pools or in this case a puddle. Knowing that temperature is often a major factor in their competitiveness and the rotifers’ recorded locales, I suspect that Polyarthra species must have a dormant phase during which they diapause or hibernate when the water warms up during the summer (especially in the case of P. dolichoptera, which prefers water temperatures of only 3-5 degrees Celsius!), and this has been reflected in the seasonal changes in these rotifers’ densities. Thus I wouldn’t be surprised if this population has that ability to diapause and regenerate, but it is pretty awesome to discover a potentially never before recorded habitat for them – in which they can not only survive unfavorable changes in temperature but also desiccation.
So that’s a wrap on Polyarthra vulgaris, with a brief overview of its unique morphology, research interest and what I found interesting about it. I’ll probably have a few more posts on puddle-water microbes (and of course lots of stuff on Chlamydomonas) before I switch topics for a little while. Stay tuned.
Works cited:
Allen, A. A. (1968). Morphology of the Planktonic Rotifer Polyarthra vulgaris. Transactions of the American Microscopical Society, 87(1), 60-69. doi:10.2307/3224338
Haney, J.F. et al. “An-Image-based Key to the Zooplankton of North America” version 5.0 released 2013. University of New Hampshire Center for Freshwater Biology <cfb.unh.edu> 8 Dec 2017
Laxhuber, R. (1987). Abundance and distribution of pelagic rotifers in a cold, deep oligotrophic alpine lake (Königssee). Hydrobiologia, 147(1), 189-196.
Saunders-Davies, A. (1989). Horizontal distribution of the plankton rotifers Keratella cochlearis (Bory de St Vincent) and Polyarthra vulgaris (Carlin) in a small eutrophic lake. Hydrobiologia, 186(1), 153-156.
Stenson, J. (1983). Changes in the relative abundance of Polyarthra vulgaris and P. dolichoptera , following the elimination of fish. Hydrobiologia, 104(1), 269-273.